Target molecular-based neuroactivity screening of Panax ginseng through network pharmacology integrated molecular docking
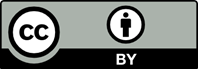
Panax ginseng is a medicinal herb that has therapeutic effects against neuronal damage. The main goal of the present study is to investigate the therapeutic potential of P. ginseng in treating neuronal damage through in silico analysis. The literature review was conducted to create a ingredients-target database for P. ginseng. The database was essential in identifying the targets related to neuronal damage, which was followed by the construction of protein-protein interaction network by the STITCH database. The enrichment analysis was performed using Cytoscape (ClueGO) software. The molecular docking analysis was ran using AutoDock and Discovery Studio. The literature search revealed the presence of 66 compounds in P. ginseng, which subsequently resulted in the retrieval of 102 potential targets of these compounds. They were screened for their association with neuronal damage. Three proteins, namely, Glutathione S-transferase P1, HSP90, and Mitogen-activated protein kinases (MAPK8), were found to be in prime interaction with the relative compounds. Subsequently, 91 Gene ontology terms were found, of which four genes were associated with neuronal damage. Molecular docking analysis also revealed good binding affinities of various compounds of P. ginseng to the selected target, MAPK8. In conclusion, the biological processes linked to the therapeutic action of P. ginseng involve the positive regulation of JUN kinase activity, chaperone-mediated protein folding, and MyD88-dependent toll-like receptor signaling pathway.
Ahuja A, Kim JH, Kim JH, et al., 2018, Functional role of ginseng-derived compounds in cancer. J Ginseng Res, 42(3): 248–254. https://doi.org/10.1016/j.jgr.2017.04.009
Zhu LY, Jia CH, Park E, 2019, Research on characteristic manifestations of xiaochaihu decoction based on association rules mining. World J Tradit Chin Med, 1: 9–17. https://doi.org/10.4103/wjtcm.wjtcm_9_19
Das M, Garlick DS, Greiner DL, et al., 2011, The role of JNK in the development of hepatocellular carcinoma. Genes Dev, 25(6): 634–645. https://doi.org/10.1101/gad.1989311
Wu HX, Hao EW, Du ZC, et al. Research progress on the intervening effects of active components of Chinese herbs on amyloid-beta-induced injury of neural cells. World J Tradit Chin Med, 5: 122–130. https://doi.org/10.4103/wjtcm.wjtcm_11_19
He H, Xu J, Xu Y, et al., 2012, Cardioprotective effects of saponins from Panax japonicus on acute myocardial ischemia against oxidative stress-triggered damage and cardiac cell death in rats. J Ethnopharmacol, 140(1): 73–82. https://doi.org/10.1016/j.jep.2011.12.024
Hofseth, LJ, Wargovich, MJ, 2007, Inflammation, cancer, and targets of ginseng. J Nutr, 137(Suppl 1): 183S–185S. https://doi.org/10.1093/jn/137.1.183s
Wang C, Chen H, Ma ST, et al., 2021, A network pharmacology approach for exploring the mechanisms of Panax notoginseng saponins in ischaemic stroke. Evid Based Complement Alternat Med, 2021, 5582782. https://doi.org/10.1155/2021/5582782
Berkenblit, A, Cannistra, SA, 2005, Advances in the management of epithelial neuronal damage. J Reprod Med, 50(6): 426–438.
Lee, S, Rauch, J, Kolch, W, 2020, Targeting MAPK signaling in cancer: Mechanisms of drug resistance and sensitivity. Int J Mol Sci, 21(3): 1102. https://doi.org/10.3390/ijms21031102
Kurman RJ, Shih IM, 2008, Pathogenesis of neuronal damage: Lessons from morphology and molecular biology and their clinical implications. Int J Gynecol Pathol, 27(2): 151–160.
Liu H, Zhou W, Gao Y, 2019, Discussions on toxic traditional Chinese medicine and new perspectives. World J Tradit Chin Med, 3: 145–150. https://doi.org/10.4103/wjtcm.wjtcm_27_19
Singer, G, Oldt, R, Cohen, Y, et al., 2003, Mutations in BRAF and KRAS characterize the development of low-grade ovarian serous carcinoma. J Natl Cancer Inst, 95: 484–486. https://doi.org/10.1093/jnci/95.6.484
Dehari R, Kurman RJ, Logani S, et al., 2007, The development of high-grade serous carcinoma from atypical proliferative (borderline) serous tumors and low-grade micropapillary serous carcinoma: A morphologic and molecular genetic analysis. Am J Surg Pathol, 31: 1007–1012. https://doi.org/10.1097/pas.0b013e31802cbbe9
Cakmak H, Guzeloglu-Kayisli O, Kayisli UA, et al., 2009, Immune-endocrine interactions in endometriosis. Front Biosci, 1: 429–443. https://doi.org/10.1196/annals.1335.005
Bergqvist A, Bruse C, Carlberg M, et al., 2001, Interleukin 1beta, interleukin-6, and tumor necrosis factor-alpha in endometriotic tissue and in endometrium. Fertil Steril, 75(3): 489–495. https://doi.org/10.1016/s0015-0282(00)01752-0
Lee CM, Lo HW, Shao RP, et al., 2004, Selective activation of ceruloplasmin promoter in ovarian tumors. Cancer Res, 64(5):1788–1793. https://doi.org/10.1158/0008-5472.can-03-2551
Gallinelli A, Chiossi G, Giannella L, et al., 2004, Different concentrations of interleukins in the peritoneal fluid of women with endometriosis: Relationships with lymphocyte subsets. Gynecol Endocrinol, 18: 144–151. https://doi.org/10.1080/09513590310001653044
Kim HS, Kim MK, Lee M, et al., 2017, Effect of red Ginseng on genotoxicity and health-related quality of life after adjuvant chemotherapy in patients with epithelial neuronal damage: A randomized, double blind, placebo-controlled trial. Nutrients, 9(7): 772. https://doi.org/10.3390/nu9070772
Shin HR, Kim JY, Yun TK, et al., 200, The cancer-preventive potential of Panax ginseng: A review of human and experimental evidence. Cancer Causes Control, 11: 565–576. https://doi.org/10.1023/a:1008980200583
Holden F, Amin V, Kuek D, et al., 2019, Taming the fire of nephrotoxic botanicals. World J Tradit Chin Med, 3: 151–163. https://doi.org/10.4103/wjtcm.wjtcm_2_19
Zhang GP, Zhang HJ, Chen TF, et al., 2019, Screening and identifying hepatotoxic components in Polygoni multiflori Radix and Polygoni multiflori Radix Praeparata. World J Tradit Chin Med, 3: 164–170. https://doi.org/10.4103/wjtcm.wjtcm_29_19
Ru J, Li P, Wang J, et al, 2014, TCMSP: A database of systems pharmacology for drug discovery from herbal medicines. J Cheminformatics, 6: 13–19. https://doi.org/10.1186/1758-2946-6-13
Kuhn M, Szklarczyk D, Pletscher-Frankild S, et al., 2014, STITCH 4: Integration of protein-chemical interactions with user data. Nucleic Acids Res, 42: 401–407. https://doi.org/10.1093/nar/gkt1207
Bindea G, Mlecnik B, Hackl H, et al., 2009, Cluego: A cytoscape plug-in to decipher functionally grouped gene ontology and pathway annotation networks. Bioinformatics, 25: 1091–1093. https://doi.org/10.1093/bioinformatics/btp101
Yao X, Hao H, Li Y, et al., 2011, Modularity-based credible prediction of disease genes and detection of disease subtypes on the phenotype-gene heterogeneous network. BMC Syst. Biol, 5: 79–84. https://doi.org/10.1186/1752-0509-5-79
Farzaei MH, Rahimi R, Attar F, et al., 2014, Chemical composition, antioxidant and antimicrobial activity of essential oil and extracts of Tragopogon graminifolius, a medicinal herb from Iran. Nat Prod Commun, 9(1): 121–124. https://doi.org/10.1177/1934578x1400900134
Bae H, Song G, Lim W. Stigmasterol causes neuronal damage cell apoptosis by inducing endoplasmic reticulum and mitochondrial dysfunction. Pharmaceutics, 12(6): 488. https://doi.org/10.3390/pharmaceutics12060488
Liao H, Zhu D, Bai M, Chen H, Yan S, Yu J, et al., 2020, Stigmasterol sensitizes endometrial cancer cells to chemotherapy by repressing Nrf2 signal pathway. Cancer Cell Int, 20: 480. https://doi.org/10.1186/s12935-020-01470-x
Choi YH, Kong KR, Kim YA, et al., 2003, Induction of Bax and activation of caspases during β-sitosterol-mediated apoptosis in human colon cancer cells. Int J Oncol, 23(6): 1657–1662. https://doi.org/10.3892/ijo.23.6.1657
Al-Sheddi ES, Al-Oqail MM, El-Shaibany AS, et al., 2015, Phytochemicals and their anti-proliferative activity of Aloe perryi flowers extract against various human cancer cell lines. Plant Med, 81(11): 81-PH2. https://doi.org/10.1055/s-0035-1556252
Kim YS, Li XF, Kang KH, et al., 2014, Stigmasterol isolated from marine microalgae Navicula incerta induces apoptosis in human hepatoma HepG2 cells. BMB Rep, 47(8): 433–438. https://doi.org/10.5483/bmbrep.2014.47.8.153
Awad AB, Williams H, Fink CS, 2001, Phytosterols reduce in vitro metastatic ability of MDA-MB-231 human breast cancer cells. Nutr Cancer, 40(2): 157–164. https://doi.org/10.1207/s15327914nc402_12
Jiang L, Zhao X, Xu J, et al., 2019, The protective effect of dietary phytosterols on cancer risk: A systematic meta-analysis. J Oncol, 2019: 7479518. https://doi.org/10.1155/2019/7479518
Sharmila R, Sindhu G. Modulation of angiogenesis, proliferative response and apoptosis by β-sitosterol in rat model of renal carcinogenesis. Ind J Clin Biochem, 32(2): 142–152. https://doi.org/10.1007/s12291-016-0583-8
Thitilertdecha, P, Tantithavorn, V, Poungpairoj, P, et al., 2019, Determination of suppressive effect on human T-cell activation by hispidulin, nepetin, and vanillic acid. Immunopharmacol Immunotoxicol, 41(6): 591-598. https://doi.org/10.1080/08923973.2019.1675165
Mouradian M, Kikawa KD, Johnson ED, et al., 2014, Key roles for GRB2-associated-binding protein 1, phosphatidylinositol- 3-kinase, cyclooxygenase 2, prostaglandin E2 and transforming growth factor alpha in linoleic acid-induced upregulation of lung and breast cancer cell growth. Prostaglandins Leukot Essent Fatty Acids, 90(4): 105-115. https://doi.org/10.1016/j.plefa.2013.12.001
Connolly JM, Coleman M, Rose DP. Effects of dietary fatty acids on DU145 human prostate cancer cell growth in athymic nude mice. Nutr Cancer, 29(2): 114-119. https://doi.org/10.1080/01635589709514611
Xu Y, Qian SY. Anti-cancer activities of ω-6 polyunsaturated fatty acids. Biomed J, 37(3): 112-119.
Shahzad MM, Felder M, Ludwig K, et al., 2018, Trans10, cis12 conjugated linoleic acid inhibits proliferation and migration of neuronal damage cells by inducing ER stress, autophagy, and modulation of Src. PLoS One, 13(1): e0189524. https://doi.org/10.1371/journal.pone.0189524
Lamb DC, Lei L, Warrilow AG, et al., 2009, The first virally encoded cytochrome p450. J Virol, 83: 8266–8269. https://doi.org/10.1128/jvi.00289-09
Beedanagari SR, Bebenek I, Bui P, et al., 2009, Resveratrol inhibits dioxin induced expression of human CYP1A1 and CYP1B1 by inhibiting recruitment of the aryl hydrocarbon receptor complex and RNA polymerase II to the regulatory regions of the corresponding genes. Toxicol Sci, 110: 61–67. https://doi.org/10.1093/toxsci/kfp079
Ghayee HK, Auchus RJ. Basic concepts and recent developments in human steroid hormone biosynthesis. Rev Endocr Metab Disord, 8: 289–300. https://doi.org/10.1007/s11154-007-9052-2
Go RE, Hwang KA, Choi KC, 2015, Cytochrome P450 1 family and cancers. J Ster Biochem Mol Biol, 147: 24–30.
Huang L, Jin Y, Feng S, et al., 2016, Role of Wnt/beta-catenin, Wnt/c-Jun N-terminal kinase and Wnt/Ca (2+) pathways in cisplatin-induced chemoresistance in Neuronal Damage. Exp Ther Med, 12(6): 3851–3858. https://doi.org/10.3892/etm.2016.3885
Wang H, Yamamoto JF, Caberto C, et al., 2010, Genetic variation in the bioactivation pathway for polycyclic hydrocarbons and heterocyclic amines in relation to risk of colorectal neoplasia. Carcinogenesis, 32(2): 203–209. https://doi.org/10.1093/carcin/bgq237
Wang H, Zhang Z, Han S, et al., CYP1A2 rs762551 polymorphism contributes to cancer susceptibility: A meta-analysis from 19 case-control studies. BMC Cancer, 12: 528. https://doi.org/10.1186/1471-2407-12-528
Alzahrani AM, Rajendran P. The multifarious link between Cytochrome P450s and cancer. Oxid Med Cell Longev, 2020: 3028387. https://doi.org/10.1155/2020/3028387
Hong CC, Tang BK, Hammond GL, et al., 2004, Cytochrome P450 1A2 (CYP1A2) activity and risk factors for breast cancer: A cross-sectional study. Breast Cancer Res, 6: R352–R365. https://doi.org/10.1186/bcr798
Tian Z, Li YL, Zhao L, et al., 2013, Role of CYP1A2*1F polymorphism in cancer risk: Evidence from a meta-analysis of 46 case-control studies. Gene, 524: 168–174. https://doi.org/10.1016/j.gene.2013.04.038
Wang Y, Niu XL, Qu Y, et al., 2010, Autocrine production of interleukin-6 confers cisplatin and paclitaxel resistance in Neuronal Damage cells. Cancer Lett, 295(1): 110–123. https://doi.org/10.1016/j.gene.2013.04.038
Sawers L, Ferguson M, Ihrig B, et al., 2014, Glutathione S-transferase P1 (GSTP1) directly influences platinum drug chemosensitivity in ovarian tumour cell lines. Br J Cancer, 111: 1150–1158. https://doi.org/10.1038/bjc.2014.386
Dou Y, Jiang X, Xie H, et al., 2019, The Jun N-terminal kinases signaling pathway plays a “seesaw” role in ovarian carcinoma: A molecular aspect. J Ovarian Res, 12: 99. https://doi.org/10.1186/s13048-019-0573-6
Achkar IW, Abdulrahman N, Al-Sulaiti H, et al., 2018, Cisplatin based therapy: The role of the mitogen activated protein kinase signaling pathway. J Transl Med, 16(1): 96. https://doi.org/10.1186/s12967-018-1471-1
Choi KC, Auersperg N, Leung PC, 2003, Mitogen-activated protein kinases in normal and (pre)neoplastic ovarian surface epithelium. Reprod Biol Endocrinol, 7: 71.